1. Bistatic Radar
The knowledge of wind vector fields throughout the atmosphere is extremely desirable for both meteorological research and operational applications. While with conventional methods for deriving wind vector fields within precipitation, e.g., combining two Doppler weather radars (Fig. 1, monostatic dual-Doppler) at a distance between 40-80 km are quite expensive.
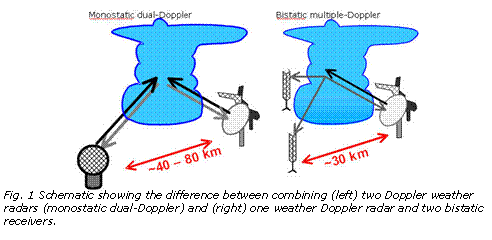
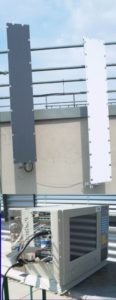
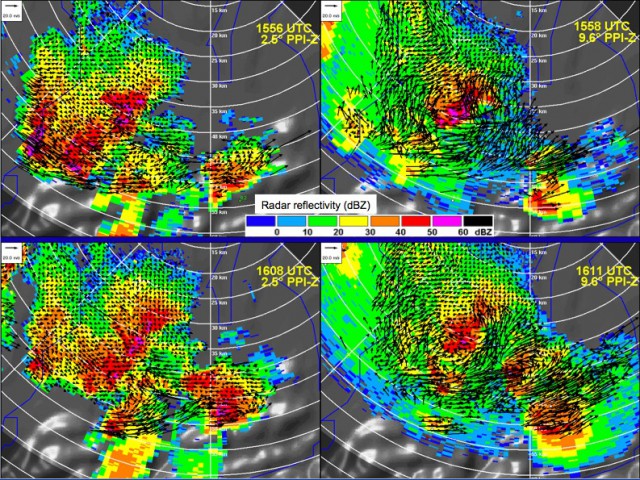
2. Dual-polarization radars
Usually the orographic environment around the radar is characterized in terms of calculating the area that is illuminated by the radar beam for a given scanning strategy and radar characteristics (Fig. 4).While numerous studies have focused on the influence of ground clutter on the quality of radar reflectivity only few investigated the effects of mountains on the quality of polarimetric measurements.
Radar reflectivity (Zh), differential reflectivity (Zdr), and specific differential phase (Kdp) measured from the operational, polarimetric weather radar located in Trappes, France, were used to examine the effects of radar beam shielding on rainfall estimation. The objective of this study is to investigate the degree of immunity of Kdp-based rainfall estimates to beam shielding for C-band radar data during four typical rain events encountered in Europe. The large effects of beam shielding on rainfall accumulation were observed for algorithms using Zh and Zdr with differences of up to ~2 dB (40%) compared to a Kdp-based algorithm over a power loss range of 0–8 dB (Fig. 5).
This analysis reveals that Zdr and Kdp are not affected by partial beam shielding. Standard reflectivity corrections based on the degree of beam shielding would have overestimated rainfall rates by up to 1.5 dB for less than 40% beam shielding and up to 3 dB for beam shielding less than 75%.
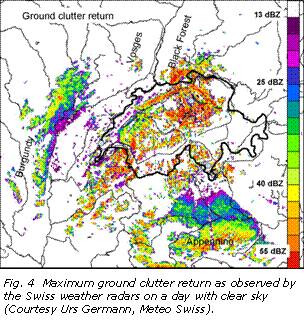
The investigation also examined the sensitivity of beam shielding effects on rainfall rate estimation to (i) axis–ratio parameterization and drop size distribution, (ii) methods used to smooth profiles of differential propagation phase (phi_dp) and estimate Kdp, and (iii) event-to-event variability. Although rainfall estimates were sensitive to drop size distribution and axis–ratio parameterization, differences between Zh- and Kdp-based rainfall rates increased independently from those parameters with amount of shielding. Different approaches to smoothing phi_dp profiles and estimating Kdp were examined and showed little impact on results.
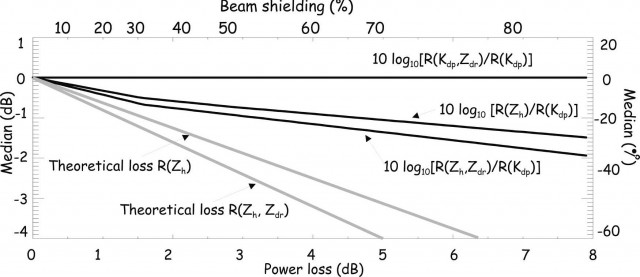